In the landscape of modern materials science, the discovery of graphene stands as a monumental achievement marking a new era of technological possibilities. Isolated for the first time in 2004 by Andre Geim and Konstantin Novoselov at the University of Manchester, graphene emerged from the realm of theoretical physics into tangible reality, challenging the conventional boundaries of materials science.
The University of Manchester has played a pivotal role in propelling graphene from an intriguing theoretical concept to a cornerstone of cutting-edge scientific research and development. By creating a culture of innovation and interdisciplinary collaboration, the institution has established itself as a global leader in graphene studies, attracting top-tier talent and investment from around the world. The university’s dedicated Graphene Engineering Innovation Centre (GEIC) and National Graphene Institute (NGI) serve as hubs for research and commercialization, bridging the gap between academic discovery and real-world applications.
The isolation of graphene by researchers at the University of Manchester is a narrative of simplicity leading to groundbreaking discovery employing a remarkably straightforward technique known as mechanical exfoliation or the “Scotch Tape Method.” By repeatedly peeling layers off graphite with simple adhesive tape, they managed to obtain flakes of graphene only one atom thick. This seemingly simple method showcased a complex and significant outcome. It also illustrated how a material that was theoretically speculated due to its unimaginable thinness and fragility to come to fruition.
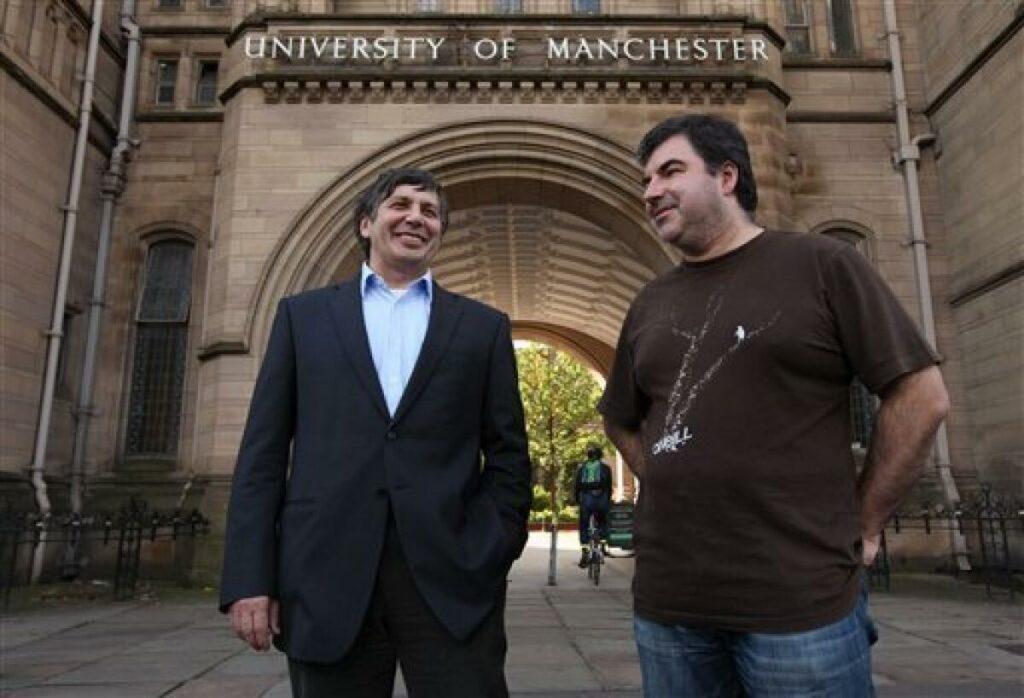
This one-atom-thick layer of carbon atoms, arranged in a two-dimensional honeycomb lattice, boasts an array of exceptional properties, including unparalleled strength, remarkable electrical conductivity, and exceptional thermal and optical characteristics. Its discovery not only earned Geim and Novoselov the Nobel Prize in Physics in 2010, but also ignited a global surge in graphene research, underscoring its potential to revolutionize industries ranging from electronics and energy storage to medical devices and beyond.
The theoretical journey to understanding graphene’s existence and properties was fraught with challenges, primarily due to the skepticism surrounding the stability of two-dimensional materials. Prior to graphene’s isolation, it was widely believed among physicists that purely two-dimensional crystals could not exist in a stable form at room temperature, as thermal fluctuations would cause them to crumble or roll up. Graphene defied this logic, existing as a stable, two-dimensional sheet of carbon atoms. Theoretical work, including thought experiments and computational modeling, played a crucial role in unraveling how graphene’s unique atomic arrangement conferred stability and extraordinary properties. These theoretical explorations entered into the realms of quantum mechanics and materials science, offering insights into the material’s remarkable characteristics.Graphene’s high electrical conductivity and mobility of charge carriers suggested potential for faster, more efficient electronic devices, while its strength and flexibility indicated new possibilities for composite materials. Additionally, its transparency and flexibility held implications for flexible display technologies and photovoltaic cells.
The inspiration into graphene research was twofold, encompassing both scientific curiosity and the anticipation of practical applications. Scientists were intrigued by the potential of a material that could revolutionize fields given its unique properties. This blend of curiosity-driven and application-oriented research underscored the multidisciplinary nature of graphene studies, propelling the scientific community to explore the material’s potential further. The allure of graphene lies not just in its remarkable physical and chemical properties, but also in the promise it holds for future innovations, making it a focal point of research efforts worldwide.
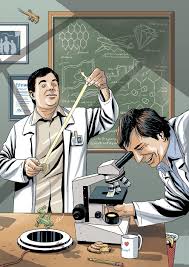
To add to its capabilities, graphene’s strength is unparalleled being about 200 times stronger than steel, yet it is incredibly lightweight and flexible! This combination opens up new possibilities for creating materials that are both durable and adaptable, a dream for industries ranging from aerospace to micro-electronics. To provide a comparison of its electrical conductivity, it is superior to that of copper! These characteristics, including its exceptional heat conductivity and optical transparency, make graphene a candidate for revolutionizing not just one, but multiple fields of technology, from creating faster, more efficient electronic devices and transparent conductive coatings to improving the performance of thermal management materials.
The impact of these findings on the scientific community and various industries has been profound and far-reaching. In the realm of electronics, graphene is being explored for use in touchscreens, light displays, and even in advanced computing technologies that could surpass the limits of silicon-based devices. The energy sector sees potential in graphene for developing more efficient solar panels, batteries with higher energy densities, and supercapacitors that could dramatically reduce charging times. Moreover, its strength and flexibility are inspiring innovations in materials science, including the development of stronger, lighter composites for vehicles, sports equipment, and construction. The discovery of graphene has not only expanded our understanding of material properties but has also sparked a global race to harness its potential, leading to significant investments in research and development. As scientists and engineers continue to unlock the full capabilities of graphene, it stands as the king of the transformative power of materials science, promising to shape the future of technology in unprecedented ways.
The University of Manchester’s work on graphene serves as a blueprint for future generations of scientists, showcasing the profound impact of a collaborative and exploratory spirit in the realm of scientific research and discovery. Their environment, characterized by an open exchange of ideas and a willingness to transcend traditional disciplinary boundaries, has proven instrumental in the discovery of graphene and its myriad potential applications. The university’s approach encourages researchers to pursue curiosity-driven inquiries where innovative solutions emerge from the confluence of diverse perspectives and expertise. Such a model of collaboration and exploration is essential for tackling complex scientific challenges and paves the way for future breakthroughs. It underscores the importance of creating research environments that are not only equipped with cutting-edge facilities but are also imbued with a culture of mutual respect, curiosity, and the shared pursuit of knowledge.
Encouragement for interdisciplinary research and the recognition of curiosity-driven inquiry as a fundamental driver of scientific advancement are key lessons from Manchester’s success story. The journey of graphene from a theoretical concept to a material with real-world applications exemplifies the power of interdisciplinary collaboration, combining physics, engineering, chemistry, and materials science. This integrative approach has not only accelerated the pace of graphene research but also broadened its potential applications, highlighting the critical role of curiosity in pushing the boundaries of what is scientifically possible. Universities and research institutions worldwide are inspired to foster environments that value and support the exploration of uncharted scientific territories, driven by a quest for understanding and innovation.
The role of thought experiments and theoretical modeling in advancing material science and engineering cannot be overstated. These intellectual tools allow scientists to explore the implications of theories and hypotheses in a conceptual realm, paving the way for physical technological innovations. Theoretical modeling, in particular, has been crucial in understanding the properties of graphene and predicting its behavior in various applications. By engaging in thought experiments, scientists can challenge existing paradigms and envision novel materials and technologies, thereby expanding the horizons of material science and engineering. As future scientists and engineers look to this example, they are reminded that a process as simple as the “Scotch Tape Method” can offer limitless potential that lies in the synergy between theoretical inquiry and practical experimentation, a synergy that continues to shape the future of science and technology.