Feynman diagrams are a pictorial representation of the mathematical expressions governing the behavior of subatomic particles. First proposed in 1948 by physicist Richard Feynman, these simple line drawings provide a powerful method for understanding and calculating the interactions between particles.
Richard Feynman was an American theoretical physicist known for his developmental contributions to quantum mechanics and quantum electrodynamics. Born in 1918 in New York City, Feynman displayed exceptional intelligence from a young age. He eventually obtained his PhD from Princeton in 1942 and joined the Manhattan Project at Los Alamos shortly thereafter, during World War II. At Los Alamos, the 26-year-old Feynman was not only one of the youngest group leaders, but also stood out for his style and personality.
Outside of physics, Feynman had a passion for art, safecracking, and the bongo drums. He was very outgoing and developed a reputation as a teller of fantastic stories and prankster at Los Alamos. Feynman was married three times, most happily to his third wife Gweneth. After an illustrious career, he passed away in 1988 at the age of 69 after being diagnosed with cancer.
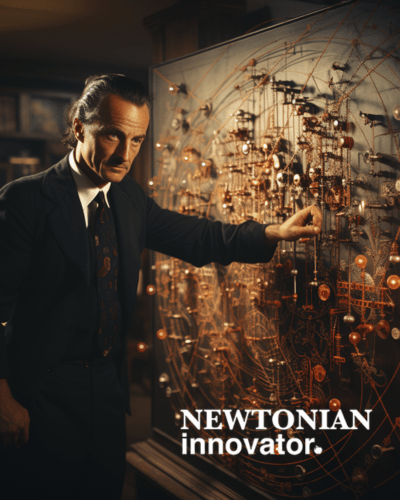
After the Manhattan Project at 31 years old, Feynman found himself in a period of introspection and uncertainty about his future research direction. He took up a position at Cornell University, but he felt a lack of motivation and direction in his work. He described this period as a time when he was “burned out” and felt he had become a “fraud” because he wasn’t producing significant research.
One day in the Cornell cafeteria, Feynman observed a student throwing a plate in the air. As the plate wobbled in the air, Feynman became curious about the motion, specifically the relationship between the plate’s wobble and its rotation. This simple observation led him to start playing with the equations of motion for the plate. While this problem had nothing to do with the cutting-edge physics he was known for, it rekindled his natural curiosity and love for physics.
Feynman was reinvigorated and began to tackle the challenges of QED (quantum electrodynamics), a theory that describes how light and matter interact. The mathematics of QED was notoriously difficult. Traditional methods involved lengthy calculations with terms that seemed to give infinite results, which technically didn’t make sense.
Feynman started to think about these interactions in a new way. Instead of getting bogged down in the complex mathematics, he visualized the interactions between particles as a series of simple diagrams. Each diagram represented a different possible interaction between particles. The diagrams simplified the process of calculating probabilities for different interactions.
While originally conceived as a thought experiment, Feynman diagrams proved to have enormous practical applications. They provide exact computations of probability amplitudes for quantum electrodynamics. This allowed Feynman to produce results accurate to four decimal places matching experimental data, a major breakthrough.
The idea was to represent complex interactions using simple, intuitive pictures. For example, an electron moving forward in time could be represented as a straight line, while a photon (a particle of light) could be represented as a wavy line. Points where the lines intersected represented interactions between the particles. By drawing all possible diagrams for an interaction and then translating these diagrams back into mathematical terms, Feynman could more easily calculate the probability of that interaction occurring.
To construct a diagram, you draw horizontal lines to denote particles traveling forward in time and vertical lines for antiparticles traveling backward in time. The position along the horizontal axis indicates progression through space-time. These particle lines can represent electrons, photons, or any other elementary particle. The lines interact when they converge at vertices, which symbolize interactions like scattering or decay. Another example is two electrons colliding and scattering. This involves two incoming electron lines meeting at a vertex and two outgoing lines diverging. The diagram encodes the momentum and energy at each stage.
Like most new scientific concepts, Feynman’s approach was initially met with skepticism, but it soon became clear that his diagrams provided a powerful tool. His diagrams provide a simple visualization of the mathematical expressions governing the interactions between subatomic particles.
Beyond quantum electrodynamics, Feynman diagrams provide approximation methods useful across modern physics. They give order-of-magnitude estimates for any particles interacting at high energies. Particle physicists rely on Feynman diagrams to model new theories like the electroweak interaction.
Feynman diagrams also aid in computing radiative corrections. As particles interact, they emit radiation that can alter the overall amplitude. Diagrams help account for these corrections which are critical for accurate predictions. The Higgs boson was discovered in 2012 after identifying radiative corrections indicating its existence. Feynman diagrams have even proven useful for understanding biology systems and phenomena in condensed matter physics like superconductivity.
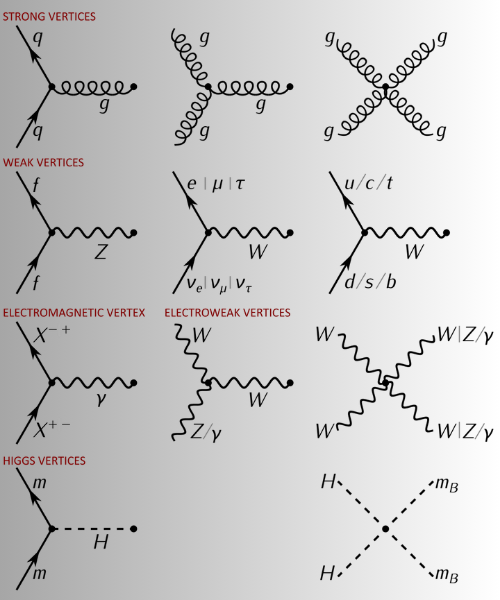
Richard Feynman exemplified the power of visual, intuitive modes of thinking to provide clarity on complex problems. His introduction of simple pictorial representations for particle interactions shows the value of thought experiments and creativity within physics. In 1949, Feynman published two groundbreaking papers using this method to formulate a theory of quantum electrodynamics. For this work, Feynman shared the 1965 Nobel Prize in Physics. The success of Feynman diagrams marked the beginning of his rise to the top ranks of physics. He went on to make seminal contributions to areas from nanotechnology to quantum computing. By finding such an elegant solution to a complex problem at an early age, the Feynman diagram thought experiment proved instrumental in advancing his storied career. After winning the Nobel Prize and becoming a celebrity scientist, Feynman went on to serve on the panel investigating the Challenger disaster in 1986.
Fast forwarding to modern times, the images produced by particle detectors at CERN (the European Organization for Nuclear Research) and other high-energy physics laboratories around the world are often interpreted and understood using the language of Feynman diagrams. When particles collide at high energies in the LHC, they can produce a plethora of other particles in complex interactions. Feynman diagrams provided a conceptual framework for understanding the particle interactions that the detectors are capturing, helping physicists visualize these interactions and calculate the probabilities of various outcomes.
Today, In an era where the complexities of scientific research can often seem insurmountable, Richard Feynman’s approach to problem-solving serves as inspiration. His ability to simplify intricate problems confirms the power of creative thinking. Today’s researchers, across all disciplines, can draw valuable lessons from Feynman’s methodology.
In a technological world, it’s easy to become overly reliant on advanced computational methods and lose sight of the foundational principles that support scientific process. Feynman’s diagrams remind us of the importance of grounding our understanding in basic concepts, of seeking clarity amidst complexity. By visualizing intricate interactions through simple line drawings, Feynman demonstrated that sometimes, the most profound insights arise from stripping a problem down to its core.
Researchers emulating Feynman’s simplicity means having the ability to take a step back from their work, to question the underlying principles, and to seek alternative perspectives. This might mean representing data visually, creating physical models, reverse engineering or even to streamline complex scenarios with the process of elimination.
Feynman’s journey highlights the importance of resilience and self-belief. In the face of skepticism from the scientific community, he remained steadfast in his convictions, trusting the elegance and simplicity of his approach. Today’s researchers, too, will inevitably face challenges and skepticism, especially when introducing novel ideas or methods. Feynman’s legacy teaches us that with genuine curiosity, a deep understanding of fundamentals, and a touch of creative flair, one can overcome the naysayers and make lasting contributions.